Synthetic diamonds have made a quantum leap in the market since 2015. Until then, lab grown diamonds have been a very minor issue in the market for the first 60 years after their first successful growth in 1953 by Asea in Sweden (monocrystalline HPHT synthetic diamond) and 1952 by the Union Carbide Corporation in the USA (polycrystalline CVD synthetic diamond). This article gives an overview of the current market situation and the identification methods and technologies, in which luminescence based testing plays a significant role (Fig. 1).
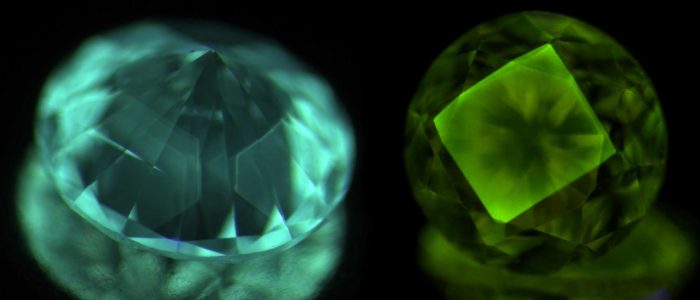
Photo: T. Hainschwang.
Background Information
Synthetic diamonds were a rare encounter in the gem market until a few years ago. Only a few companies were trying ā more or less successfully ā to market such stones; until 2010 nearly exclusively yellow and blue HPHT synthetic diamonds were commercially available, ācolorlessā goods were rarely available. The reason for this is that the HPHT presses used allowed only the growth of one stone at a time, and that the growth techniques in use resulted typically in included and off-color (yellowish) synthetic diamonds. Until 2015 such HPHT grown ānear colorlessā stones were sold at prices well above the price of comparable natural diamonds, and in consequence they were of little importance except for labs and collectors. Companies claiming that they are growing synthetic diamonds from the ashes of deceased persons were ā until then ā probably one of the few business models that were successful with synthetic diamond marketing.

The real rise of synthetic diamonds was initiated with the first growth of near colorless monocrystalline CVD synthetic diamonds by the company Apollo Diamonds (USA) in 2003; this method was found to produce mostly near colorless synthetic diamond and hence there was finally the potential to have near colorless diamonds at reasonable cost. Even though promises about immediate marketing of CVD diamonds were heard already in 2003, it took until 2010 that the first samples were actually available commercially: near colorless HPHT treated CVD synthetic diamonds were marketed by the company Gemesis, which also sold colored HPHT grown synthetic diamonds; this was the first time that near colorless synthetic diamonds became available at a price a few percent below the price of comparable natural diamond. From 2010 on things started to evolve and more and more companies started with the growth of synthetic diamond, mostly by the CVD process. In the meantime, while all the experts were betting that the CVD process would be the growth method for the future – particularly for ānear colorlessā synthetic diamonds – a company in Russia called New Diamond Technology (NDT) developed new growth procedures in new types of HPHT presses. In these new ācubic pressesā (Fig. 2) they started to grow much bigger near colorless and blue HPHT synthetic diamonds of better quality, and several stones per run, and all that at lower cost. Hence for the first time in the history of HPHT synthetic diamonds, near colorless gem-quality stones became available at a significantly lower cost than natural diamond, and in very large sizes of up to 10 cts. The cubic presses in use are manufactured in China, and not surprisingly the Chinese industry started to grow synthetic diamonds as well, but mostly in small sizes. This industry developed very rapidly and today thousands of HPHT presses in China are in use to produce near colorless melee-sized synthetic diamonds for the gem market. Unfortunately, it appears that the majority of such produced melee synthetic diamonds ends up in mixed within parcels of natural diamonds, and only a small portion is actually used in jewelry and declared as what they are. This leads to the critical situation that a very small percentage of synthetic diamonds ā mostly HPHT grown ā can be found in many parcels of colorless to near colorless diamonds today, a situation that is well known for parcels of yellow melee diamonds since at least 2010; in every single parcel of vivid yellow melee diamonds submitted to GGTL Laboratories for testing, the author has found HPHT synthetic diamonds, from 0.2 up to 35% (on average 1 to 2%). For the high-quality parcels of colorless to near colorless diamond that pass through our labs the percentage is much lower, and often parcels are free from synthetics. This is not representative for other qualities and markets though, in India the percentage is said to be significantly higher, but numbers given are not reliable.
While HPHT diamonds have spread rapidly, the growth of CVD synthetic diamond made rapid progress as well and today many companies worldwide grow single-crystal CVD synthetic diamonds for the gem market, in sizes up to more than 4 cts.
The Properties of Synthetic Diamonds
Much has been written about the properties of synthetic diamonds, thus only a short summary will be given in this work.
Synthetic diamonds are essentially structurally and chemically identical to natural diamond, but the growth which differs from the growth of natural diamonds is responsible for minor differences in properties that are characteristic for synthetic diamonds.
HPHT synthetic diamonds are grown on seed crystals in a solvent-catalyst, typically an Ni-Fe or Ni-Co alloy but recently also alternative metal alloys and even non-metallic solvent-catalyst, in order to grow larger single crystals. Commercially grown HPHT synthetic diamonds grow as type Ib, hence containing single nitrogen impurities (C centers) and in consequence being of yellow color. If colorless synthetic diamond is desired a so-called nitrogen-getter (such as Zr, Hf, Al or Ti) to get rid of the nitrogen in the growth environment through the formation of nitrides, needs to be used as well. Traces of the catalysts can generally be found in such HPHT synthetic diamonds. The growth conditions that permit rapid and efficient high quality growth result generally in crystals with dominant octahedral (111) and cube (100) faces, with additionally dodecahedral (110) and trapezohedral (113) faces (Shigley et al, 1987). These crystal habits are very different from what you expect for natural gem-quality diamonds, which are typically octahedral and more or less affected dissolution or of mixed cuboid-octahedral growth; to outline the difference between cube and cuboid faces, cube faces are flat and sharp-edged while cuboid faces are rough and irregularly edged. Cuboid faces do not exist in synthetic diamonds but are very common in natural diamonds while cube faces do practically not exist in natural diamonds but are very common in synthetic diamonds. Defects such as nitrogen, boron and nickel are distributed in different concentrations in the different growth sectors. For nitrogen as a general rule the defect distribution within the different sectors is {111} > {100} > {113} = {110}. Cobalt and nickel impurities are found only in {111} sectors, and boron shows variable incorporation but is usually highest in {111} sectors (Burns, 1990).
Since these defects either cause color such as it is the case for the C center (yellow) and boron (blue) and nickel (brown), or luminescence which is the case for boron (greenish blue PL under 220-235 nm excitation), nickel (green PL at low temperature from the 484 nm band or green PL from the S2 and S3 center at room temperature and low temperature) and cobalt (yellow to orange PL from the 545 nm center), they can be defined either microscopically or by luminescence imaging through a strongly sector-dependent appearance.
CVD synthetic diamonds are very different to HPHT synthetic diamonds, but their properties allow their distinction from natural diamond. Dislocations and growth layers by homoepitaxial growth can be visualized by luminescence imaging. In contrast to HPHT synthetic diamonds they are not grown from graphite or diamond powder within a solvent-catalyst in an HPHT press, but from a carbon containing gas such a methane under vacuum in a CVD reactor. In consequence such diamonds are generally colorless to brownish and virtually free of nitrogen (type IIa); the only impurities present in nearly all CVD diamonds besides very low concentrations of nitrogen are silicon-related defects such as the [SiV]- defect. The source for the silicon present in the plasma seems to be in most cases the quartz bell jar in the CVD reactor (Moore et al., 2015), but silicon has been added intentionally to induce gray to violet color, and according to some sources silicon is added intentionally in order to enhance crystal growth.
The Detection Methods and Technologies to Screen Synthetic Diamonds
There are a series of methods to screen diamonds for possible synthetics and others to positively identify synthetic diamonds; some of these are specifically designed for the screening of melee-sized parcels, others are part of the typical laboratory equipment used in gem testing laboratories. The screening instruments to screen (near-) colorless melee-sized diamond parcels were introduced to the market with the goal of reassuring the market, since with the appearance of melee-sized synthetic diamonds in parcels of (near-) colorless diamonds a destabilization of this market was widely feared. Today a series of instruments are available in the market that should enable diamond dealers, diamond manufacturers, jewelry and watch manufactures to screen the (near-) colorless diamonds they are using for potential synthetic diamonds. Most of these instruments are fully automatic āblack boxesā, some instruments are simple imaging systems, and others ā such as the GGTL DFI Deep UV Laser+ System ā are laboratory instruments redesigned for the use of diamond screening.
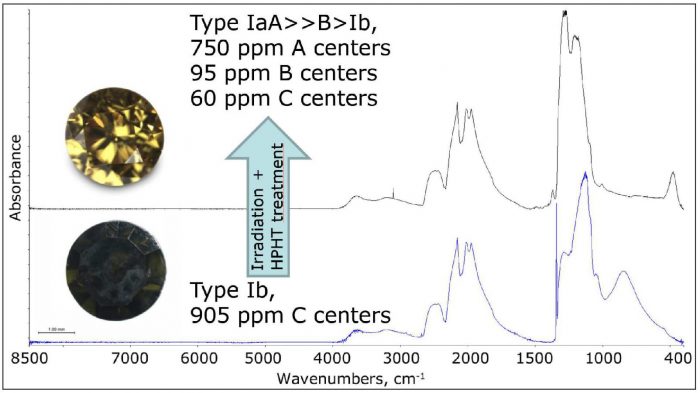
The Most Important Laboratory Techniques for the Identification of Synthetic Diamonds
Infrared Spectroscopy
In a seriously operating gem testing laboratory single diamonds (i.e., not melee) are always tested by infrared absorption spectroscopy as a first step to determine whether they could be synthetic, treated synthetic, natural or treated natural diamonds. With the necessary knowledge of defects in natural diamonds, one can get a lot of important information by infrared spectroscopy, not only the mere type determination. While commercial as-grown synthetic diamonds are typically type IIa ([near-] colorless), type Ib (yellow), and type IIb (blue), the type Ib samples can be modified via higher growth temperature and via HPHT treatment into type Ia. Via irradiation followed by HPHT treatment nearly all single nitrogen can be aggregated into A aggregates, B aggregates, N3 centers and even platelets can be formed (Fig. 3). Thus nearly all types with the exception of dominantly type IaB can occur for synthetic diamond. Due to the fact that while nitrogen aggregates by HPHT treatment, simultaneously some of the nitrogen aggregates are split up into C centers again, hence it is ā until today ā impossible to completely decolorize an originally type Ib synthetic (or natural) diamond and create a pure type Ia diamond without residual C centers. Low nitrogen type Ib diamonds can be aggregated far enough to get to only slightly colored samples, the best colors currently reported are K color or lower.
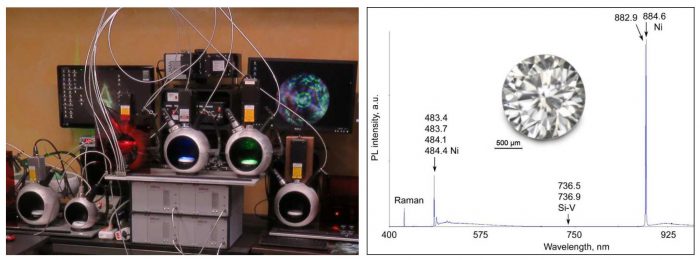
Photoluminescence Spectroscopy
Combined with infrared spectroscopy, photoluminescence spectroscopy is the preferred method to positively identify a diamond as being natural or synthetic. Here the sample to be tested are kept at the temperature of liquid nitrogen (77K, -196°C), then excited with an intense light source ā typically a laser ā in order to record the luminescence emitted by the diamond with a spectrometer (Fig. 4, left). By this method very low concentrations of defects can be detected that will characterize a diamond as being natural or synthetic. Compared to all other spectroscopic methods, this is by far the most sensitive one. For as-grown HPHT synthetic diamonds specific nickel defects such as the 484 nm center (483.4, 483.7, 484.1, 484.4 nm quadruplet) and the 884 nm center (882.9, 884.6 nm doublet), silicon defects like the [SiV] center (736.5, 736.9 nm doublet) (Fig. 4, right), and chromium impurities from most likely synthetic ruby inclusions (692.0, 693.4 nm doublet) (Iakoubovskii and Adriaenssens, 2002) or the 544.4 nm cobalt center (541.1, 542.9, 544.4 nm triplet) are characteristic. Many of the (near-) colorless HPHT synthetic diamonds have an extremely low defect content, sometimes no defects whatsoever are detectable, no matter what laser excitation used.
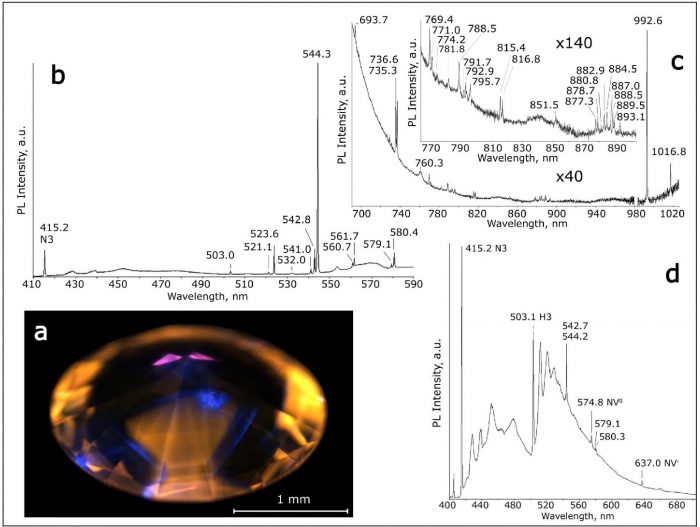
In post-growth annealed colored synthetic diamonds a multitude of peaks can often be detected; they vary depending on whether the stones were grown from Ni-Fe or Co-Fe solvent-catalyst. While the PL of Co-Fe synthetics is still mainly characterized by the 544.4 nm cobalt center ā but the PL being much more intense than in untreated samples (Fig. 5) ā the PL of Ni-Fe synthetics changes dramatically into strong green PL from the S2 and S3 centers. Depending on the laser excitation, many other Ni-related centers can be detected, but deliberating on these would exceed the scope of this article. It is interesting to note that the HPHT treatment of such yellow synthetic diamonds creates zones that contain significant concentrations of N3 centers: strong blue PL from the 415.2 nm N3 center, sometimes combined with the H3 center at 503.1 nm, can be detected (Fig. 5d).
The PL of CVD synthetic diamonds is dramatically different to the PL of HPHT synthetic diamonds, the only defect in common is the silicon vacancy defect [SiV]-, a defect thought to be unique to CVD synthetic diamond. The [SiV]- defect was detected by the author in HPHT synthetics only since 2010 and it appeared commonly in HPHT synthetics from 2014 on (DāHaenens-Johansson et al., 2014).
As-grown CVD synthetic diamonds are characterized by a large quantity of sharp bands, the most prominent being the 467 nm center, the NV0 and NV- center, the [SiV]- center doublet at 736.5/736.9 nm plus sharp peaks at 562.8, 563.3 nm and a doublet at 596.5 nm and 597.1 nm (Fig. 6, left).
When CVD synthetic diamonds are HPHT annealed in order to remove the brownish color that many of the commercially grown samples exhibit, the spectra change strongly: from orange-red PL from the NV centers, the PL changes to blue to green to violet PL from various broad bands in the range from 450 to 530 nm, with or without the NV centers. Superposed on these large bands, a large quantity of sharp peaks can be found, and finally the [SiV]- center remains detectable (Fig. 6, right). In the PL spectra of HPHT processed CVD synthetic diamonds a very weak N3 center can sometimes be detected.

Luminescence Imaging
A very powerful technique to visually distinguish natural diamonds from their synthetic counterparts is luminescence imaging. As evident from PL spectroscopy with its many defects that are detectable, many defects and their distribution can be visualized by luminescence imaging. Depending on what defect is to be visualized, different excitation bands need to be used; for most synthetic diamonds the best excitation wavelength is between 220 and 235 nm, but CVD diamonds also other wavelengths are useful. Specific instruments for luminescence imaging are the deep UV imaging system DiamondViewTM and commercially available fluorescence microscopes, which are generally limited to long wave UV excitations. The GGTL DFI Deep UV Laser+ luminescence microscope and spectroscopy system is an exception, as it offers diverse excitations from 220 nm all the way to 405 nm to observe luminescence in gemstones. Shown in figure 7, left is the Mega-DFI Deep UV Laser+ laboratory prototype ā an oversize and overpowered version of the commercially available DFI System, and on the right an image of a natural type Ia diamond under the deep UV excitation of this system. The fluorescence of natural diamonds shows specific distributions that tend to be irregular or whenever regular then it shows typical geometric patterns (Fig. 7, right); very rarely the fluorescence of natural diamond appears homogenous. The fluorescence of HPHT synthetic diamonds often shows a strongly sectored appearance (Fig. 8), while the fluorescence of CVD synthetic diamonds generally appears very homogenous (Fig. 9), and growth features can only be seen under certain angles and close-up observations. The color of luminescence is orange to red from the NV centers for as-grown CVD synthetic diamonds (Fig. 9, right) and shows a large range of colors depending on the excitation wavelength used for HPHT treated CVD synthetic diamond. Under longwave excitation the luminescence color typically ranges from blue to green to violet to purple (Fig. 9, left). After excitation by the shorter wavelengths of UV the vast majority of near colorless HPHT synthetic diamonds show strong and persisting phosphorescence induced by their boron content, while this is comparatively rare for CVD diamonds.
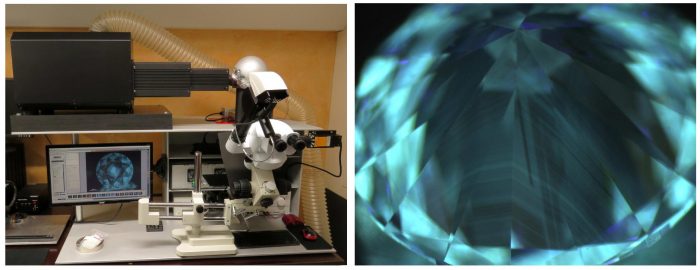
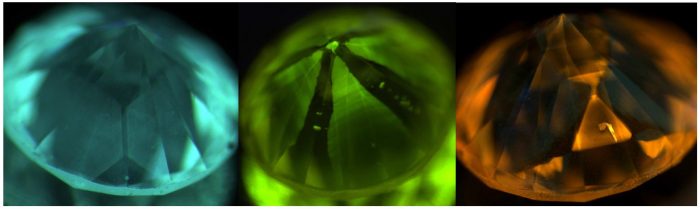
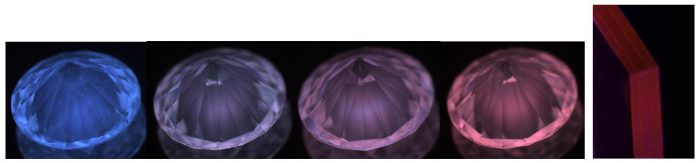
Screening Systems to Identify (Near-) Colorless Melee-Sized Synthetic Diamonds in Parcels
Automatic Screening Systems
There are a couple of fully automated screening systems in the market that permit the screening of (near-) colorless diamond parcels typically in the size range between 1 mm and 3 mm. Currently they only work for round diamonds and have variable throughput per hour, varying from a few hundred to several thousand stones screened per hour.
The machines are based on a few techniques including the following: UV transparency, Raman spectroscopy, PL spectroscopy and as the most recent technique added PL lifetime spectroscopy.
By testing UV transparency, stones that are potentially type IIa and IIb will be removed from parcels. The problem with this technique is that low nitrogen diamonds and type IaB diamonds are also transparent to the used UV radiation. In consequence, quite a lot of natural non-IIa/non-IIb diamonds are also in the batch of rejected diamonds (Fig. 10).
Raman spectroscopy is added on such machines in order to distinguish diamond from its imitations such as CZ, YAG, GGG, moissanite etc. Raman lines in a laser excited spectrum represent laser light that is inelastically scattered by the vibrations of the molecules and atoms of a material, and the resulting lines are directly dependent of the composition and crystal structure of a material. Hence a Raman spectrum is like a fingerprint of a material and is used to identify all gem materials.
PL spectroscopy can be used to distinguish a very large percentage of natural diamonds from their synthetic counterparts; the percentage approaches 100% when performed at 77K.
Very little is known about the efficiency and precision of PL lifetime spectroscopy for the distinction of natural and synthetic diamonds, the future will show how useful this technique proves to be.
Most of these machines are good for pre-screening because there is generally a non-neglectable amount of stones removed from parcels that need to be tested further at a laboratory in order to verify if they are natural or not. Even with a certain percentage of rejected diamonds, such systems are very helpful to work through large quantities of melee diamonds since they can work 24 hours per day. The danger of such automatic systems is that they are black boxes and that a new type of synthetic diamond could potentially fool such a machine and unless somebody double-checks parcels, such stones would remain unnoticed for a very long time.
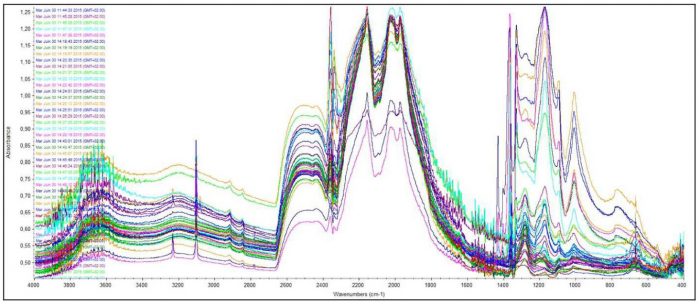
Phosphorescence-based Screening Systems
Since by far most (near-) colorless synthetic diamonds that are currently found in parcels of melee-sized diamonds are HPHT synthetic, several simple imaging systems have been developed that excite the diamonds with short wave UV light, then turn off the light and record an image to see whether diamonds phosphoresce or not. Phosphorescent diamonds are to be removed as suspected synthetics. While such a system is actually quite practical and efficient, there are several issues: first of all ā it works only for HPHT synthetic diamonds and only for very few CVD synthetic diamonds. Hence if you want to be sure there are no synthetics in a parcel, these systems are useless. Then there are HPHT synthetic diamonds that exhibit no phosphorescence under the used excitation. Next, the technique is useless for diamond imitations, and last but not least there are natural diamonds that exhibit phosphorescence. In conclusion, such phosphorescence-based screening systems are good to get rid of part of the HPHT synthetics in a parcel, but cannot be used to be sure there are no synthetic diamonds ā and imitations – left in a parcel.
Non-Automated Modified Laboratory Equipment for Diamond Screening
The DFI Deep UV Laser+ fluorescence imaging and spectroscopy system is a fluorescence microscope that has been modified and enhanced for the screening of diamonds in all sized, shapes, loose and mounted in jewelry. It is non-automated, hence needs a technician, and is based on fluorescence imaging via microscope and camera plus Raman and photoluminescence spectroscopy. The diamond parcels can be screened at room temperature and at 77K, hence the system enables the unambiguous identification of virtually all diamonds. With this system fluorescence imaging, photoluminescence and Raman spectroscopy are all performed at the same time, hence while observing the luminescence of a sample one can simultaneously verify the PL/Raman spectrum. With the DFI system a trained technician can run more than 2000 diamonds per hour.
Concluding Remarks
With the recent advent of synthetic diamonds the market has been exposed to an enormous challenge. The main problem of synthetic diamonds sold at prices significantly lower than natural diamonds is that such stones are misrepresented as natural diamonds. In a market where by far most diamonds from a certain size on are accompanied by a laboratory report, the bigger diamonds are not an important problem: such ālargerā sizes of synthetic diamonds are easily identified when submitted to a laboratory.
The problem faced by the market is the mixing of synthetic diamonds in parcels of natural diamonds; this problem is a reality for melee-sized yellow diamonds since about 2010 and for (near-) colorless diamonds since 2014, and ever since the problem has reached the (near-) colorless diamond market, solutions are being searched. The quantity of synthetic diamonds grown has increased very significantly in the past two years and today particularly the Chinese market produces very large quantities of mostly melee-sized (near-) colorless HPHT synthetic diamonds. CVD diamonds are being produced in significant quantities by several companies worldwide as well, but almost no melee-sized material is marketed, only stones above 0.20 ct are generally available.
The many options available today to get melee diamonds either tested at a gemological laboratory or to screen them using one of the commercially available screening machines permit the trade to protect itself from the threat imposed by undeclared synthetic diamonds.
About the author:
Dr. Thomas Hainschwang (thomas.hainschwang@ggtl-lab.org) is director and research scientist at the Liechtenstein branch of GGTL Laboratories in Balzers, Liechtenstein.
References
Burns, R. C., Cvetkovic, V., Dodge, C. N., Evans, D. J. F., Rooney, M. L. T., Spear, P. M., & Welbourn, C. M. (1990) Growth-sector dependence of optical features in large synthetic diamonds. Journal of Crystal Growth, Vol. 104, No. 2, pp. 257-279.
DāHaenens-Johansson, U. F., Moe, K. S., Johnson, P., Wong, S. Y., Lu, R., Wang, W. (2014) Near colorless HPHT synthetic diamonds from AOTC Group. Gems & Gemology, Vol. 50, No. 1, pp. 2-14.
Iakoubovskii, K., Adriaenssens, G. J. (2002). Comment on āEvidence for a Fe-related defect centre in diamondā. Journal of Physics: Condensed Matter, Vol. 14, No. 21, p. 5459.
Moore, S. L., Vohra, Y. K. (2015) Nitrogen and silicon defect incorporation during homoepitaxial CVD diamond growth on (111) surfaces. In MRS Proceedings (Vol. 1734, pp. mrsf 14-1734). Cambridge University Press.
Shigley, J. E., Fritsch, E., Stockton, C. M., Koivula, J. I., Fryer, C. W., Kane, R. E., Hargett, D. R., Welch, C. W. (1987). The gemological properties of the De Beers gem-quality synthetic diamonds. Gems and Gemology, Vol. 23, No. 4, pp. 187-206.
Request your copy of Rivista Italiana di Gemmologia or buy a yearly subscription
Article by Thomas Hainschwang, published on Rivista Italiana di Gemmologia #1, May 2017.